Research
Our purpose is “Understanding the structure-property relationships of materials and realizing Material Design”
A huge amount of time and effort has been spent on material development. However, as society continues to change dynamically and speedy, a need for more accurate and rapid material development than ever before is greatly increasing. If we can realize Material Design by understanding the correlation between atomic/electronic structure and functional expression, we will be able to dramatically accelerate material development. In order to realize the material design, it is necessary to elucidate the atomic and electronic structures of local regions that are responsible for the function arising, and to understand the mechanisms from the results. We conducts research with the aim of ``realizing Materials Mesign'' by making full use of first-principles calculations, information science , and atomic-level characterization.
Our research subjects are the followings:
Materials research using materials informatics
In recent years, data science has been attracting attention as a fourth paradigm, and research on "materials informatics," which uses data science for materials research, is progressing worldwide. In our laboratory, we are building artificial intelligence using information science methods (informatics) and working on the following research themes.
1) Development of a method to quickly determine the interface structure
2) Development of a method to predict interface physical properties
3) Development of a physical property measurement method that combines spectral analysis and machine learning
4) Development of artificial intelligence technology for high-speed calculation of excited states
5) Development of a method to predict surface properties in advance
For example, using our method, calculations that would take 22 years can be completed in just 3 hours. Furthermore, by using artificial intelligence technology, we have succeeded in obtaining new knowledge that even specialized researchers were unable to obtain until now.
Keywords: materials informatics, data-driven, artificial intelligence, interface, surface, spectrum
“Prediction of the Ground State Electronic Structure from Core-loss Spectra of Organic Molecules by Machine Learning”
PY. Chen, K. Shibata, K. Hagita, T. Miyata, and T. Mizoguchi
J. Phys. Chem. Lett., 14 (2023) 4858-4865.
“Learning excited states from ground states by using an artificial neural network
S. Kiyohara, M. Tsubaki, and T. Mizoguchi, npj Comp. Mater., 6 (2020) 68-1-6. here
“Machine learning applications for ELNES/XANES “
T. Mizoguchi and S. Kiyohara, Microscopy 69 (2020) 92-109. here
“Prediction of interface structures and energies via virtual screening”
S. Kiyohara, H. Oda, T. Miyata, and T. Mizoguchi, Science Adv., 2 (2016) e1600746-1-7. here
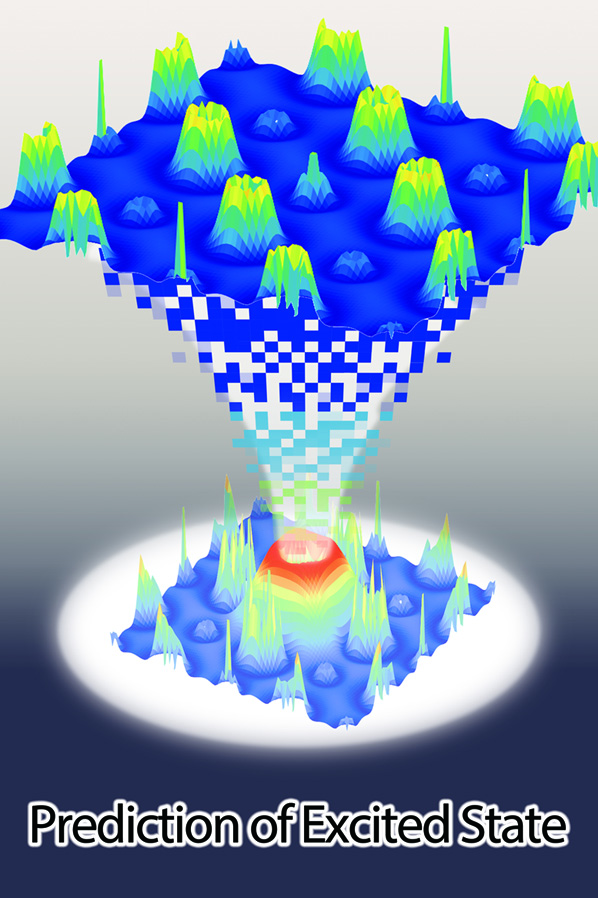
Understanding structure-function relationships in energy-related materials
In order to achieve carbon neutrality, the development of high-performance power generation and storage materials is essential. In this laboratory, we use first-principles calculations, machine learning, and atomic-level analysis to analyze energy-related materials such as solar cell materials, secondary battery materials, ionic conductors, and superconductors, and analyze the effects of lattice defects such as interfaces and dopants. Our research aims to understand the relationship between structure and function using atomic and electronic structure analysis and data-driven analysis.
Keywords: solar cell materials, fuel cell materials, ionic conduction band, superconductor
“Possible New Graphite Intercalation Compounds for Superconductors and Charge Density Wave Materials: Systematic Simulations with Various Intercalants Using a van der Waals Density Functional Method”
N. Kawaguchi, K. Shibata, and T. Mizoguchi, J. Phys. Chem. C, 127 (2023) 9833-9843.
“A valence state evaluation of a positive electrode material in a Li-ion battery with first-principles K- and L-edge XANES spectral simulations and resonance photoelectron spectroscopy”
K. Kubobuchi, M. Mogi, M. Matsumoto, T. Baba, C. Sato, T. Yamamoto, T. Mizoguchi, H. Imai, J. Appl. Phys., 120, 142125-1-13 (2016)
“The atomic structure, band gap, and electrostatic potential at the (112)[1-10] twin grain boundary of CuInSe2”
H. Yamaguchi, H. Hiramatsu, H. Hosono, and T. Mizoguchi, Appl. Phys. Lett. , 104, 153904-1-5 (2014).
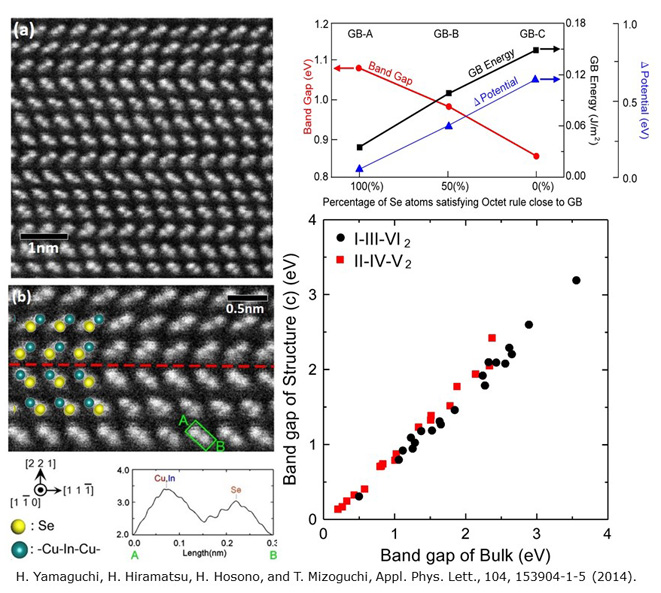
Lattice defect formation and dynamics in semiconductor and ceramic materials
Due to the dramatic improvement in computational speed in recent years, it is becoming possible to calculate the electronic state of systems consisting of several hundred atoms based on first principles without using parameters. In our laboratory, we use the latest first-principles calculations to study the formation behavior and dynamics of defects, dopants, lattice defects on surfaces, interfaces, etc. in advanced materials such as semiconductors and ceramics.
Keywords: semiconductors, ceramics, two-dimensional compounds
“A defect formation mechanism induced by structural reconstruction of a well-known silicon grain boundary”
YS. Xie, K. Shibata, and T. Mizoguchi, Acta Mater., 250 (2023) 118827-1-11.
“The influence of neighboring vacancies and their charge state on the atomic migration of LaAlO3”
T. Yamamoto and T. Mizoguchi, Appl. Phys. Lett., 102 (2013) 211910-1-4.
“Defect energetics in LaAlO3 polymorphs: A first principles study”
T. Yamamoto and T. Mizoguchi, Phys. Rev. B, 86 (2012) 094117.
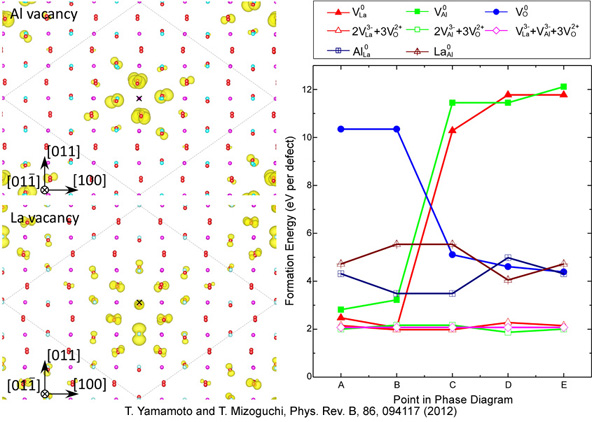
Material design of glasses, liquids, and soft materials using atomic resolution measurement
Glass, liquids, gases, and soft materials are widely used in daily life and industrial activities. The physical properties of these materials are caused by local atomic structure disturbances and changes in dynamic behavior, but their complex structures make local structural analysis extremely difficult. In this laboratory, we are developing methods to analyze glass, liquids, gases, and soft materials with high spatial resolution by making full use of transmission electron microscopy, and applying them to real materials. So far, we have observed the behavior of each atom in a liquid in real space, observed the dynamic behavior of gas molecules with high spatial resolution, and observed high-temperature phase separation phenomena in glass in real space and in real time. We have succeeded in elucidating this quantitatively.
Keywords: glass, ionic liquid, electrolyte, fuel cell, surfactant, soft material, gas
“Revealing Spatial Distribution of Al-Coordinated Species in a Phase-Separated Aluminosilicate Glass by STEM-EELS”
K. Liao, A. Masuno, A. Taguchi, H. Moriwake, H. Inoue, and T. Mizoguchi, J. Phys. Chem. Lett., 11 (2020) 9637–9642. here
“In situ observation of the dynamics in the middle stage of spinodal decomposition of a silicate glass via scanning transmission electron microscopy” K. Nakazawa, S. Amma, and T. Mizoguchi, Acta Mater. 200 (2020) 720-726. here
“Real-space analysis of diffusion behavior and activation energy of individual monatomic ions in a liquid”
T. Miyata, F. Uesugi, and T. Mizoguchi, Science Advances, 3 (2017) e1701546-1-5. here
“Estimation of the molecular vibration of gases using electron microscopy”
H. Katsukura, T. Miyata, M. Shirai, H. Matsumoto, and T. Mizoguchi, Scientific Reports, 7 (2017), 16434-1-9. here
“An estimation of molecular dynamic behavior in a liquid using core-loss spectroscopy”
Y. Matsui, K. Seki, A. Hibara, T. Mizoguchi, Scientific Reports, 3 (2013) 3503-1-7. here
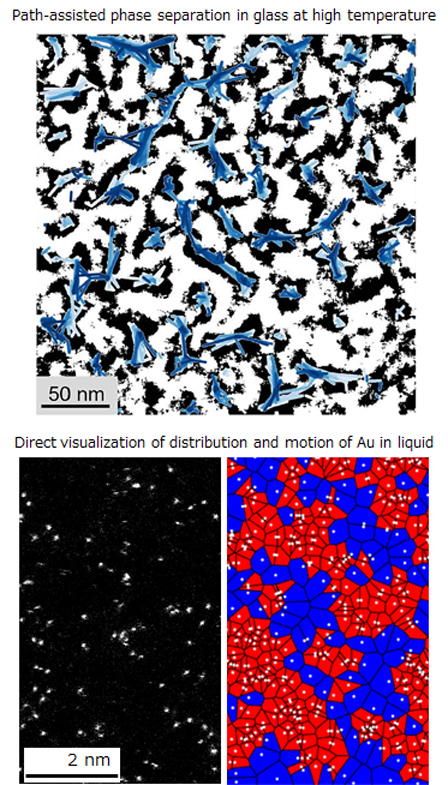
Atomic resolution material analysis using the “ultimate analysis method”
By combining spherical aberration scanning transmission electron microscopy (STEM) and electron beam energy loss spectroscopy (EELS), it is possible to obtain information about the atomic and electronic structure of materials at atomic resolution, making it possible to obtain "The Ultimate Analysis". It is a material analysis method so powerful that it is called In our laboratory, we are conducting research aimed at establishing material design by applying such "ultimate analytical methods" to advanced materials such as artificial superlattices and optical fibers.
Keywords: amorphous material, artificial superlattice, Li-ion battery cathode material, solar cell material
“Nanoscale Investigation of Local Thermal Expansion at SrTiO3 Grain Boundaries by Electron Energy Loss Spectroscopy”
K. Liao, K. Shibata,and T. Mizoguchi, Nano Letters, 21 (2021) 10416-10422
“Controlling interface intermixing and property of SrTiO3 based superlattices”
T. Mizoguchi, H. Ohta et al., Adv. Funct. Mater. 21, (2011) 2258–2263.
“Atomic Scale Identification of Individual Lanthanide Dopants in Optical Glass Fiber”
T. Mizoguchi et al., ACS Nano, 7 (2013) 5058-5063.
“Site dependence and Peak assignment of YBa2Cu3O7 OK ELNES”
T. Mizoguchi et al.,Phys. Rev. B, 77 (2008) 024504-1-5.
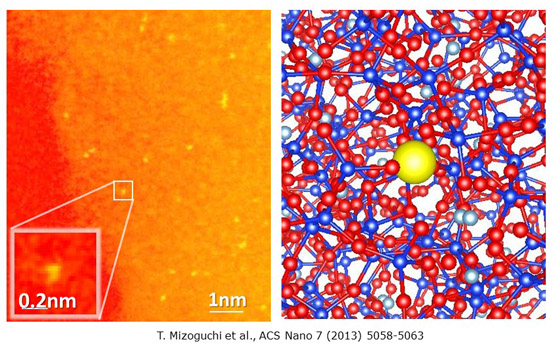
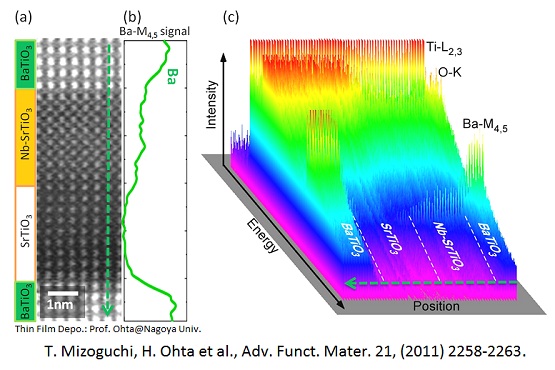
Establishment of a first-principles calculation method for core electron excitation spectra
Electron energy absorption edge near-edge fine structure (ELNES) and X-ray absorption edge fine structure (XANES) are both core excitation spectra that occur when core electrons transition to unoccupied orbitals. ELNES and XANES are powerful material analysis methods with high spatial and temporal resolution and detection sensitivity, but theoretical calculations of spectra using first-principles calculations are essential for spectrum interpretation. Our laboratory is working on the development of a theoretical calculation method for ELNES/XANES, and is currently conducting research aimed at "establishing a theoretical calculation method for all structures, all elements, and all absorption edges."
Keywords: core electron excitation spectroscopy, ELNES, XANES, single particle, exciton, many electrons
“Basics and Applications of ELNES calculation” [Invited Review]
H. Ikeno and T. Mizoguchi, Microscopy, 66 (2017) 305–327.
“Excitonic, vibrational, and van der Waals interactions in electron energy loss spectroscopy” [Invited Manuscript]
T. Mizoguchi, T. Miyata, and W. Olovsson, Ultramicroscopy, 180 (2017) 93-103.
“Strong excitonic interactions in the oxygen K-edge of perovskite oxides”
K. Tomita, T. Miyata, W. Olovsson, and T. Mizoguchi, Ultramicroscopy, 178 (2017) 105-111.
“Core–Excitonic Interaction in Sodium L2,3 Edge Structure Investigated Using the Bethe-Salpeter Equation”
K. Tomita, T. Miyata, W. Olovsson, and T. Mizoguchi, J. Phys. Chem. C, 120 (2016) 9036 -9042.
“Theoretical ELNES: one particle and many particle calculations” [Invited Review]
T. Mizoguchi, W. Olovsson, H. Ikeno, and I. Tanaka, Micron 41 (2010) 695–709
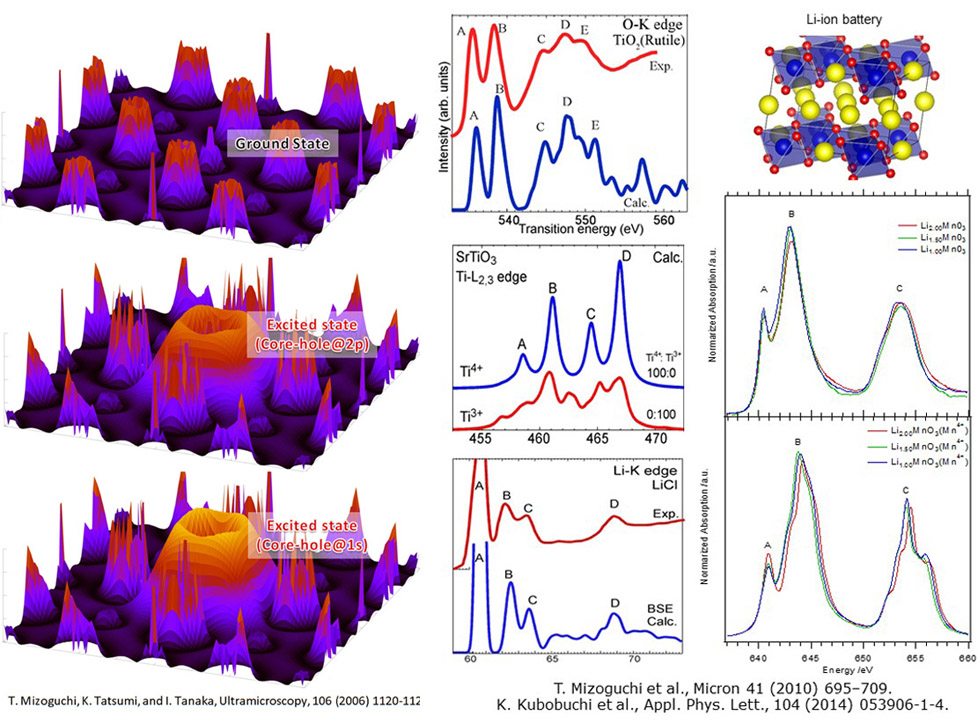